Startup Project
3d Etching of Silicon and Glasses
Three-dimensional (3D) etching of materials by plasmas is an ultimate challenge in microstructuring applications. A method is proposed to reach a controllable 3D structure by using masks in front of the surface in a plasma etch reactor in combination with local magnetic fields to steer the incident ions in the plasma sheath region towards the surface to reach 3D directionality during etching and deposition. This effect can be controlled by modifying the magnetic field and/or plasma properties to adjust the relationship between sheath thickness and mask feature size. Since the guiding length scale is the plasma sheath thickness, which for typical plasma densities is at least 10s of microns or larger, controlled directional etching and deposition target the field of microstructuring, e.g. of solids for sensors, optics, or microfluidics. In this proof-of-concept study, it is shown that E x B drifts tailor the local sheath expansion, thereby controlling the plasma density distribution and the transport when the plasma penetrates the mask during an RF cycle. This modified local plasma creates a 3D etch profile. This is shown experimentally as well as using 2d3v Particle-In-Cell/Monte Carlo collisions simulation.
Animated result of the PIC simulation covering one RF cycle for a bias voltage of -100 V and a magnetic field of 80 mT.
Further details see Arxiv: Plasma sheath tailoring by a magnetic field for three-dimensional plasma etching
Complete projects
Development of innovative thermal insulating layers and coating processes for injection molding tools
In order to produce high-quality injection molds, the optimization of the molding process parameters plays a crucial role. The application of thermal barrier coatings onto the molding block slightly slows down the cooling of the plastics injected into it. This increases the energy efficiency of the injection process, without compromising the quality of the manufactured parts. To produce these barrier coatings metal organic chemical vapor deposition (MOCVD) combined with plasma activation is explored (PECVD). Significant improvements are expected regarding the achievable growth rates of the coatings (>500 nm/h) as well as the required layer thicknesses (<30 μm). Injection molds for plastics processing require coatings for corrosion protection and thermal insulation. For this, a low permeation of the coating material is necessary to avoid the contact of tool surfaces with corrosive media and thus extend their lifetime. The project investigates the insulating effect of deposited zirconium oxide ceramic coatings. The development and characterisation of deposited ceramic layers will be the main focus of this project. Measurements via the 3w method will be used to perform in-situ measurements of the thermal conductivity of the layers.
Videos
A video in German presenting the deposition chamber can be found here:
Completed Projects C7 SFB-TR 87
Surface processes in the interaction of high-performance plasmas with HPPMS target surfaces and plastics
In a particle beam experiment, elementary plasma processes on surfaces are mimicked by sending quantified beams of atoms or ions to metal or plastic surfaces. By this, the production of secondary electrons, the reactive sputtering and layer growth is directly being investigated. Furthermore, the plasma activation of plastic surfaces, barriers and membranes will be analysed. In the in-vacuo XPS-HPPMS system, parameters of a HPPMS plasma can be linked to a XPS analysis of the target surface. Using grafted HPPMS targets, the spatial transport of metal atoms in the HPPMS plasmas is investigated. Different Al-Cr-Ti composite and powder metallurgically manufactured targets will be sputtered in reactive Ar/O2 gas mixture and then analysed. Plasma treatments with varying parameters are performed for different substrates and the respective effect of the plasma on the substrate surface is analyzed using XPS.
Videos
A video in German presenting the particle beam experiment can be found here:
A video in German presenting XPS chamber can be found here:
Funding
The work is funded by the German Science Foundation (DFG), SFB-TR 87, project C7
Completed Projects B2 SFB TR 87
Controlling the permeation of nanocomposite materials
Development of barrier/membrane coatings from silicon oxides with superior mechanical properties (internal stress, porosity, roughness, hardness), specific material composition (studied via FTIR, XPS), and excellent ductility (by embedding silicon nanoparticles in the SiOx matrix). The aim is to systematically investigate the parameter space for the production of nanocomposite layers as a balance between nanoparticle concentration, matrix layer material (SiOx, SiOCH, a-C:H, a-Si:H) and layer thickness. It is expected that a low density of nanoparticles will lead to a reduction of the stress in those layers.
Videos
A video in German presenting the multi chamber can be found here:
A video in German presenting ICP plasmas can be found here:
Funding
The work is funded by the German Science Foundation (DFG), SFB-TR 87, project B2
Completed projects A5 SFB TR 87
High Power Impulse Magnetron Sputtering (HIPIMS)
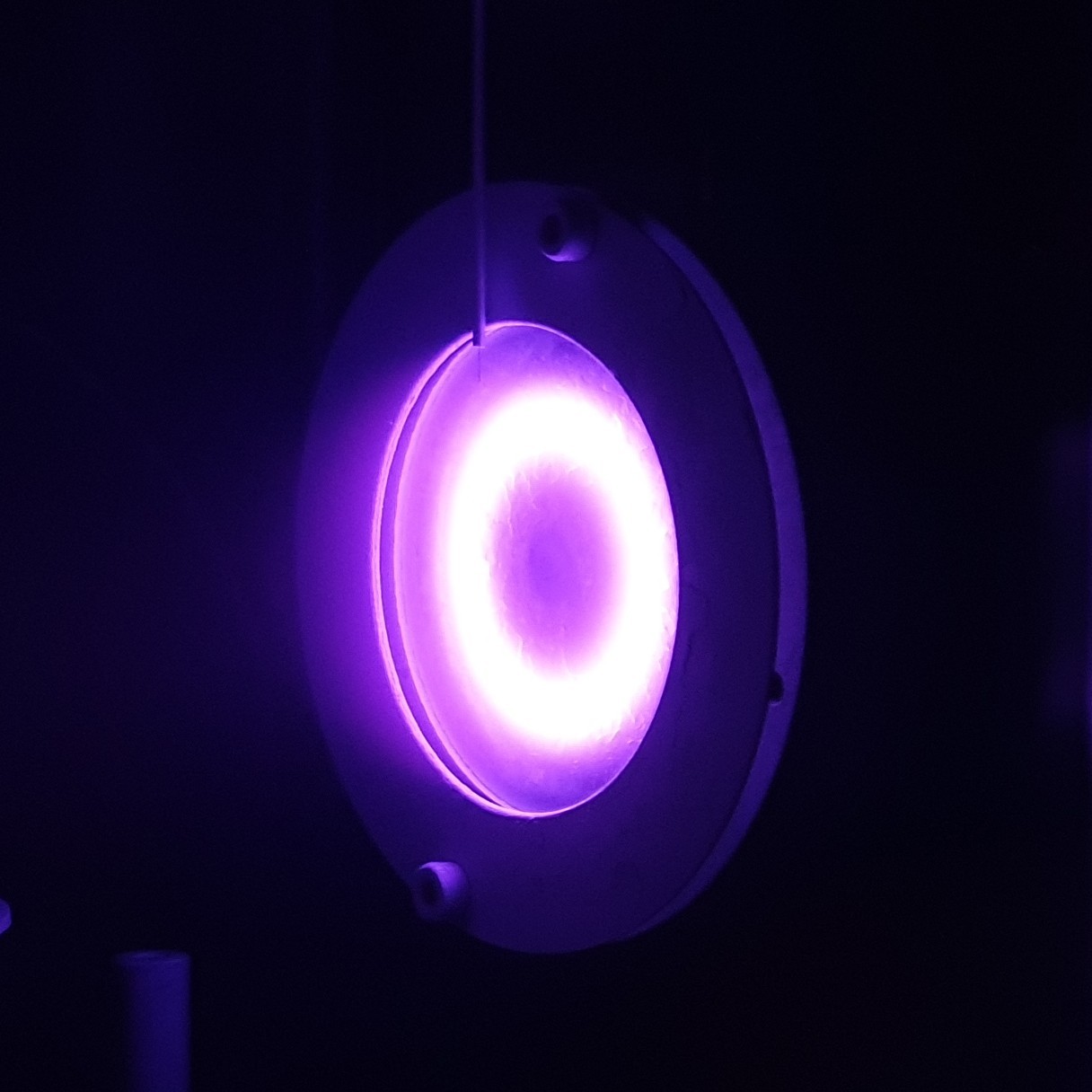
Magnetron sputtering is a widely used physical vapor deposition (PVD) technique. In magnetron sputtering, permanent magnets are placed behind a piece of metal, a so-called target. The magnetic field confines electrons to the region close to the target, where they can efficiently ionize the working gas (usually argon). A negative voltage is applied to the target, which will attract working gas ions towards the target. These ions hit the target with such high kinetic energy that they remove atoms from the target surface on impact. This process is called sputtering. Sputtered target atoms move through the discharge towards a workpiece, where they form a coating.
High power impulse magnetron sputtering (HiPIMS) is a relatively recent variation where short high voltage pulses are applied to the target instead of a continuous voltage. The result is the formation of very dense transient plasmas with a high fraction of ionized species, improving the quality of the deposited coatings in terms of density, adhesion, and hardness. However, depositing a coating of comparable thickness takes longer with HiPIMS than with traditional magnetron sputtering.
Within the frame of the project DFG TR87, our goal is to understand the physical process inside these HiPIMS plasmas with a particular focus on finding pathways to improve the deposition rate. We employ a wide variety of diagnostic methods, such as time and spatially resolved optical emission spectroscopy, a collection of different electric and magnetic probes, and energy-resolved mass spectrometry. These plasma diagnostics are combined with surface characterization methods such as x-ray photoelectron spectroscopy, which we use to characterize the surface chemistry of the deposited films or the target.
Spokes
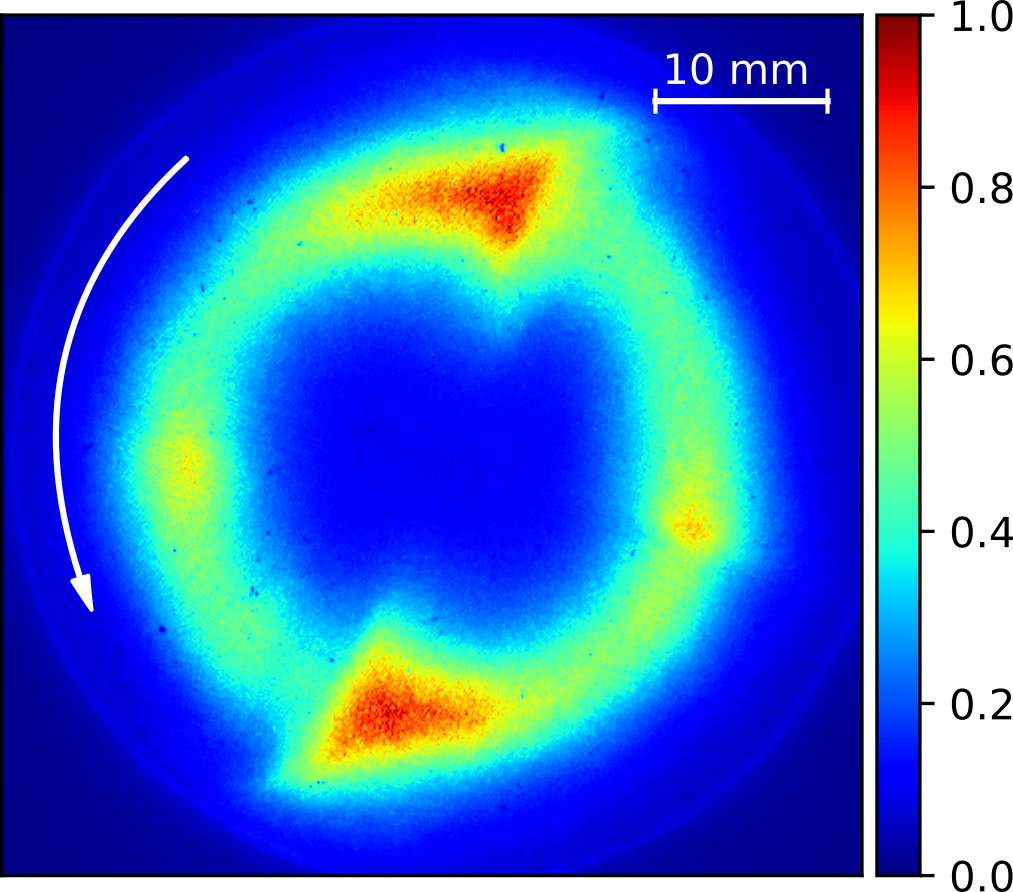
While the plasma torus formed by the magnetic field configuration (see figure 1) seems homogeneous to the naked eye, distinct structures can be observed with a fast camera (figure 2). These structures, so-called spokes, rotate along the plasma torus with velocities in the order of 10 km/s. Their increased brightness indicates a region of elevated electron density or temperature, which has profound consequences for the confinement of electrons in the magnetic trap and possibly the ion transport towards the workpiece. As such, these spokes might offer a pathway for optimizing the deposition rate of HiPIMS discharges. However, understanding these structures has proven very difficult, which is mostly due to the lack of quantitative measurements. Such measurements are very challenging since spokes emerge stochastically at random positions and times during each discharge pulse. This means that synchronization between the diagnostic tool and the spoke movement is required. Additionally, the diagnostic tool has to be very quick to reliably capture the fast spoke structure. Our goal is to perform quantitative measurements of spokes to create a solid understanding of the physical processes that create and maintain these structures. Based on this knowledge, we aim to manipulate these structures to optimize the deposition rate of HiPIMS discharges.
Videos
Here is a spoke video:
A video in German presenting HiPIMS plasmas can be found here:
Funding
The work is funded by the German Science Foundation (DFG), SFB-TR 87, project A5